China's NVIDIA to Debut on A-Share Market!
In a packed auditorium, a figure clad in a sleek black leather jacket, exuding an undeniable aura of strength, stepped onto the stage. Holding a GPU (graphics processing unit) in his hand, he was the embodiment of modern technology’s entrepreneurial spirit. This individual was not the widely recognized Jensen Huang of NVIDIA but rather a man who once served as his key lieutenant—Zhang Jianzong. Currently helming a company that has gained recognition as the “Chinese NVIDIA,” Zhang is now at the forefront of the graphics processing revolution with his venture, Moole Technology.
On November 13, a notable development emerged from the Chinese securities watchdog's official website, announcing Moole Technology’s registration for guidance and due diligence with the Beijing Securities Regulatory Bureau, signifying the commencement of its public listing process on the A-shares market. This move not only underscores the ambition of Moole Technology but also highlights its crucial role in the burgeoning sphere of domestic GPU technology, an area often overshadowed by international titans.
Advertisement
What makes Moole Technology particularly captivating is its essence, reminiscent of NVIDIA's ethos, earning it the moniker of “China’s NVIDIA.” Zhang Jianzong's credentials are impressive; he spent fifteen years with NVIDIA, where he was a crucial part of building and expanding its presence in China. Prior to forming Moole, in 2005, Zhang transitioned from a managerial position at HP to NVIDIA, climbing to the role of global vice president and general manager for the Chinese market. His leadership was pivotal in the establishment and development of a robust GPU ecosystem in China, transforming it into one of NVIDIA's most significant global markets.
By 2008, NVIDIA held less than 50% of the GPU market in China. However, by the time Zhang departed in 2020, that figure had risen above 80%. This substantial legacy laid the groundwork for Zhang's entrepreneurial pursuits. As tensions grew with the United States tightening its sanctions on Chinese tech companies, Zhang took the plunge into entrepreneurship, putting his vast experience into practice.
In 2020, with an all-star team composed of talents with backgrounds from NVIDIA, Microsoft, Intel, AMD, and ARM, he founded Moole Technology in Beijing, focusing on the research and development of high-performance domestic GPU chips and related products. The company's rapid ascent can be likened to the fervor of Chinese speed.
Within just 100 days of its establishment in February 2021, Moole Technology finished two rounds of funding totaling hundreds of millions, with renowned investors like Shenzhen Capital Group, Sequoia Capital China Fund, and GGV Capital among the backers. That same year, in November, Moole proudly announced the completion of its first fully functional domestic GPU development after an impressive 300 days. Unlike many other domestic GPU efforts, this chip was fully capable of running applications in AI and video decoding while also supporting 3D graphics, living up to its name as a fully functional GPU.
As months passed, Moole Technology launched several innovations, including the full-functional GPU chips “Suddi” and “Chunxiao,” as well as China’s first gaming graphics card, the MTT S80, and the Yuan-computing all-in-one machine, MCCX. By September 2023, they unveiled a new generation of fully functional GPU chips named “Quyuan,” accompanied by the AI training and inference integration card MTT S4000 and the Moole Technology KUAE (Kuaer) thousand card intelligent computing cluster. Their sustained rapid development is a testament to their success, effectively creating a comprehensive product line extending from chips to graphics cards to clusters.
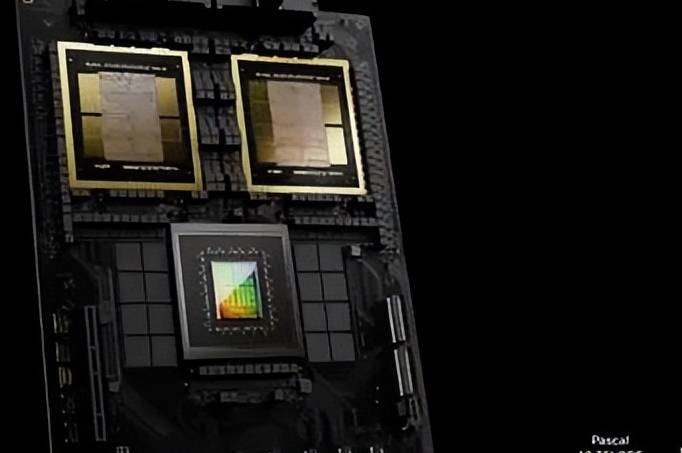
According to the National Intellectual Property Administration, by October 2024, Moole Technology had secured 425 authorized patents, solidifying its stature as the leader among Chinese GPU companies in terms of patent authorization numbers. These patents cover critical technology areas, including processor architecture design, AI applications, driver software design, and GPU computing clusters. With such a strong portfolio, the industry anticipates that Moole Technology might emerge as the next NVIDIA.
However, the path to becoming the “next NVIDIA” poses significant challenges. “At least survive for ten years; that’s our short-term goal,” Zhang Jianzong once noted, indicating his long-term vision. He emphasized a culture within the company that promotes speed, saying they must always strive for faster innovation to adapt and respond to rapid changes in policies and technologies. Zhang highlighted that the U.S. was tightening its technology export bans against China, further complicating the landscape.
On October 17, 2023, Moole Technology found itself on the U.S. Department of Commerce's “entity list,” a significant setback. Amid this turmoil, Zhang addressed his employees, expressing that the entire domestic GPU and AI chip sector had been adversely affected by this situation, denoted as “Event 1017.” However, he reinforced the commitment to their mission of delivering the best fully functional GPUs in China, asserting that nothing would deter their resolve.
Zhang outlined four avenues for striving ahead: First, to accelerate independent research and innovation. The company lead in authorized patent numbers is commendable, yet he recognizes the need for continued effort toward their goals. Second, focusing on creating high-quality products with the software team aiming to double performance efficiency to enhance competitive capabilities. Third, promoting applications' practicality in various sectors, ensuring their ecosystem remains robust. Lastly, he emphasized building an efficient team dedicated to core research and development.
Despite their momentum, the company faced formidable external constraints that complicate their aspirations. For instance, the U.S. government's actions in reaching out to TSMC, compelling it to cease shipments of 7-nanometer or more advanced AI chips to clients in mainland China starting November 2024, poses a real threat to the progression of China's GPU chip industry.
As the environment grows increasingly perilous, Moole Technology has accelerated its push for an IPO to secure cash flow and navigate the market's volatility. Other companies such as SiYu Technology and Biran Technology have also initiated listing processes. These companies are not just buoyed by private investments; they have the backing of state-owned enterprises as well, underscoring the significance of capital in this fiercely competitive landscape.
Looking ahead, the challenges are substantial. Despite numerous contenders seeking to dethrone NVIDIA, including tech giants like AMD and Intel, the reality remains that no one has yet emerged at a scale comparable to NVIDIA. Recent reports state that NVIDIA holds an overwhelming 98% of the market share in AI chips for data centers, while AMD and Intel lag far behind at a mere 1.2% and less than 1%, respectively.
Consequently, many firms in China, akin to Moole Technology, face pressing questions about their survival amidst a tumultuous market landscape exacerbated by geopolitical tensions and stringent technological constraints. The narrative of success shared by Moole Technology is one of grit, ambition, and a genuine quest to innovate within the world's most competitive and rapidly evolving tech sector, and only time will tell how this story unfolds amidst an ongoing race for supremacy in the semiconductor landscape.
Comment